As fast and sharp as can be:
data transfer via satellite
© DLR
Today, social and technical progress is digital. Given increasing data volumes, transmission rates must constantly be increasing as well. Worldwide and even beyond.
At any place all over the world, communication satellites give us access to ever-faster internet with extremely high data rates. For communications, Industry 4.0, Big Data and the mobility of the future on Earth. However, meteorologists, authorities and disaster management services depend on weather, climate and Earth observation data from space as well. With innovative laser satellite technology, transmission of these data volumes is improved – today with data rates from 1.8 to 10 Gbit/s, in the future in the terabit range.
The solution: light waves instead of radio waves – rapid data transmission via laser
So far, data are transmitted to Earth “the classic way“ via radio waves. However, radio frequencies are limited, and the existing capacities must be distributed. Given the increasing data traffic, this band width limit will soon be reached. The solution: transmission via light waves. What is working on Earth with light in the fibre optic cable will also work via laser light between satellite and, for example, ground station. Germany is in the lead for this technology. The laser revolution from Germany developed by Tesat has been in operation since 2002, first as a communication technology between the TerraSAR-X und NFIRE. For the first time, a 5.6-Gbit/s two-way communication between satellites became possible – and that between two satellites that are a) each flying at a speed of 25,000 kilometres per hour and b) on their way in different orbits. Therefore, EDRS, Europe’s data highway in space, uses laser technology: laser terminals on communication satellites serve as relay station for research satellites that also have a laser terminal for data transmission on board.

Megaconstellations enable internet
from space – everywhere
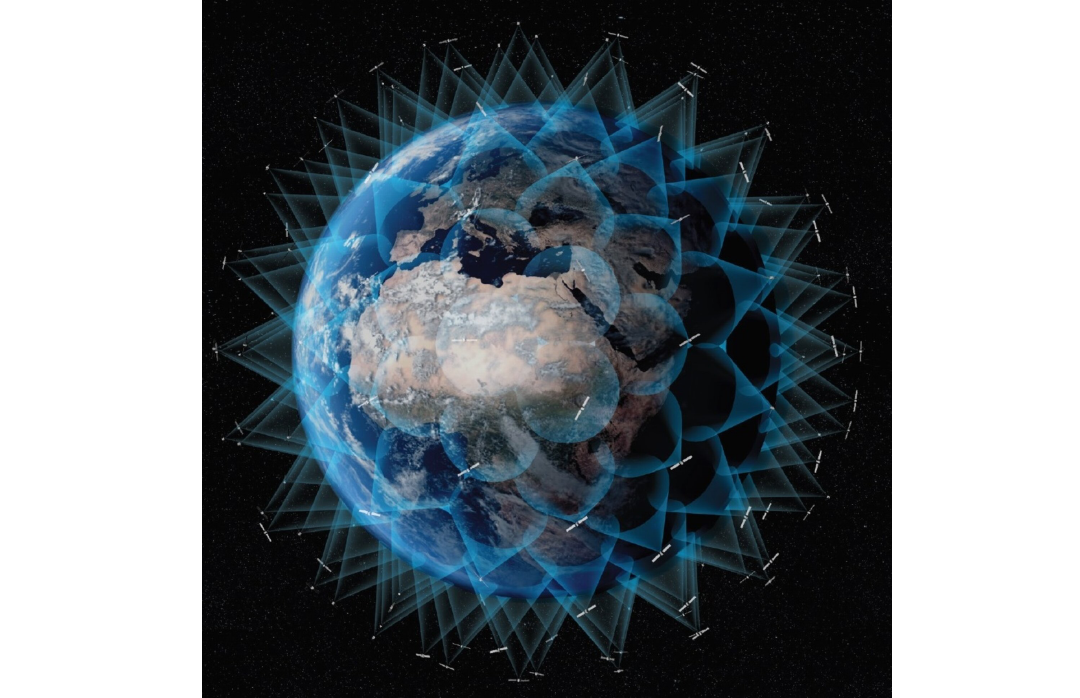
© ESA-Science Office
Internet at any place across the world, without cable, without mobile phone network? Megaconstellations of communication satellites are the future.
Internet via satellite is either provided by a few communication satellites in a high orbit of approx. 36,000 kilometres (GEO), with constellations of up to 50 satellites in a medium orbit of approx. 8,000 kilometres (MEO) such as O3b mPower by SES – or with future megaconstellations consisting of up to thousand minisatellites each in a low orbit of approx. 550 kilometres (LEO). These megaconstellations such as Starlink by SpaceX have the advantage of easier data reception since the satellites are closer to Earth. The data rates are high, too: Starlink promises 1 Gbit/s. For covering North America alone, approx. 500 satellites are required. To ensure that the satellites – and thus the users – of these megaconstellations can exchange information with a high data rate, the satellites are to communicate with each other by laser. Many more megaconstellations are planned: from Telesat, Amazon, or Hongyan.
Laser communication for quantum cryptography
© ESA - European Space Agency
Laser communication is capable of even more. For communication to be secure, it has to be encrypted. But how can these keys be safely created and distributed? With quantum technologies and satellites. Quantum keys (QKD) usually consist of polarised light particles. In case of “attempted interception“ one must hook up to the transmission, but this causes the physical properties of the particles to change. The attempted interception always becomes apparent – the key destroys itself. This is what makes the transmission that secure. For transmission of the keys, however, light must be exchanged between transmitter and receiver. Via fibre optic cable, the coverage is currently limited to approx. 150 kilometres. This does not apply to quantum key satellites with laser. This issue is presently worked on: for example, there is the QuNET initiative of the federal government – one partner being DLR – and for the satellite segment the ESA project SAGA.
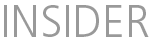
1.8 Gbit/s from satellite to satellite –
and to Earth
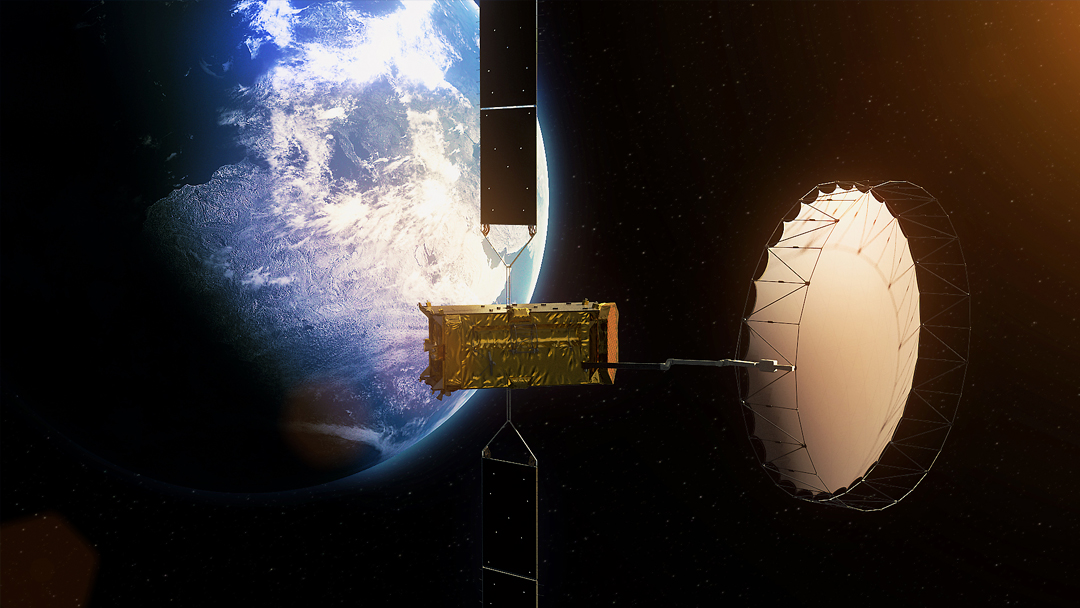
© ESA/Corvaja
Since 2013, the communication satellite Alphasat I-XL has been orbiting the Earth in a geostationary orbit. With a weight of six tons and a wingspan of the solar panels of four metres, it is the hitherto largest communication satellite. Revolutionary: on Alphasat, a laser communication terminal built by Tesat from Backnang is installed that enables
data rates of up to 1.8 Gbit/s. Via this fast data connection, it serves as a relay station:
fast-flying Earth observation satellites of the Sentinel family at a flight altitude of about
700 kilometres transmit their huge data volumes by laser “upwards” to Alphasat
Ka band with up to 600 Mbit/s. For transmitting the data, radio transmission is used since radio waves are less impaired by the Earth’s atmosphere than laser beams. The reception of the data takes place at the German Aerospace Center in Oberpfaffenhofen. The corresponding antenna features a diameter of 7.3 metres. The test run confirms: the technology is working as planned. This paves the way for the European laser satellite relay system EDRS.
The Alphasat platform was developed within the framework of an ESA-ARTES programme and is operated by Inmarsat Ltd. from London.
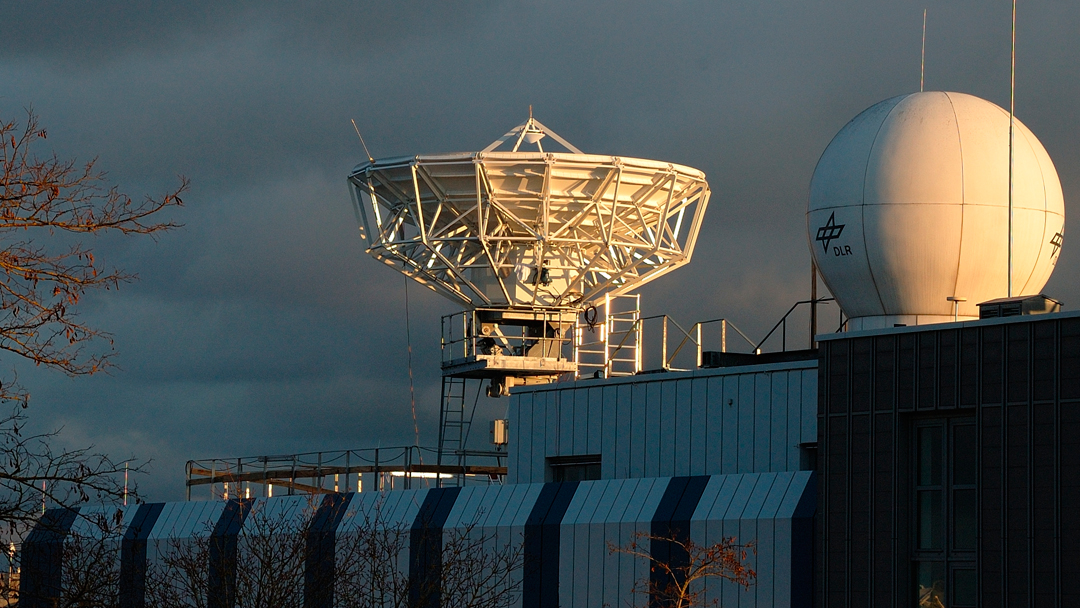
© DLR
© Tesat-Spacecom
EDRS, the data highway in orbit
The European Earth observation programme Copernicus comprises six missions and started in 2014, most missions are own Sentinel satellites. Each of the missions will continuously deliver data, presumably about six terabytes per day. This would equal over 555 Mbit/s in 24-h continuous operation.
If one uses a ground station for the reception of sensor data from Earth observation satellites in low orbit, there is only a timeframe of 10 minutes for receiving the data: at a flight altitude of 700 kilometres and a speed of 28,000 kilometres per hour, such a satellite simply is not in the visual range of a ground station for long. If not all the sensor data can be transmitted to the ground during this fly-over, the data has to be stored onboard the satellite and can only be transmitted to the ground during the next fly-over of the ground station. The waiting time can amount to up to 90 minutes, it can be even longer though. In cases where first responders have to be provided with images of an earthquake or a flooding disaster, this is not acceptable.
© Airbus DS GmbH 2015
The satellite relay network EDRS (European Data Relay System) provides a remedy that is based on laser communication. Around-the-clock reception of images from Earth observation satellites is now a reality. This “SpaceDataHighway“ receives data from satellites flying in a low orbit at high speed via laser and transmits them via radio in the Ka band (26–40 gigahertz) to the respective ground station.
With the help of this technology, Earth observation satellites can transmit their data without interruption to relay satellites in a geostationary orbit – and from there to the ground station. This is particularly important for time-critical applications like, for example, after natural disasters.
EDRS has been jointly developed by ESA and Airbus. It is operated by Airbus in Ottobrunn. EDRS has been in operation since November 2016, with EDRS-A as “subtenant“ on the Eutelsat 9B satellite. Number two, EDRS-C, was launched into the geostationary orbit in July 2019. At present, the users are the Sentinel satellites of series 1 and 2. With the launch of two additional geostationary satellites that are connected via laser – positioned above the Atlantic and the Pacific Ocean – a data relay system could emerge that is capable of providing the users with data from Earth observation satellites in real time – worldwide.
On board: technology from Backnang
The 1.8-Gbit/s laser communication terminals (LCTs) on board the satellites come
from Tesat-Spacecom in Backnang. The data rate is stable over a distance of
45,000 kilometres, that is the distance from Earth observation satellite to relay satellite in the geostationary orbit. Over smaller distances, 10 Gbit/s are possible – like from Earth to the ISS. Especially interesting for smaller satellites: a miniaturised version weighing just 300 grammes.
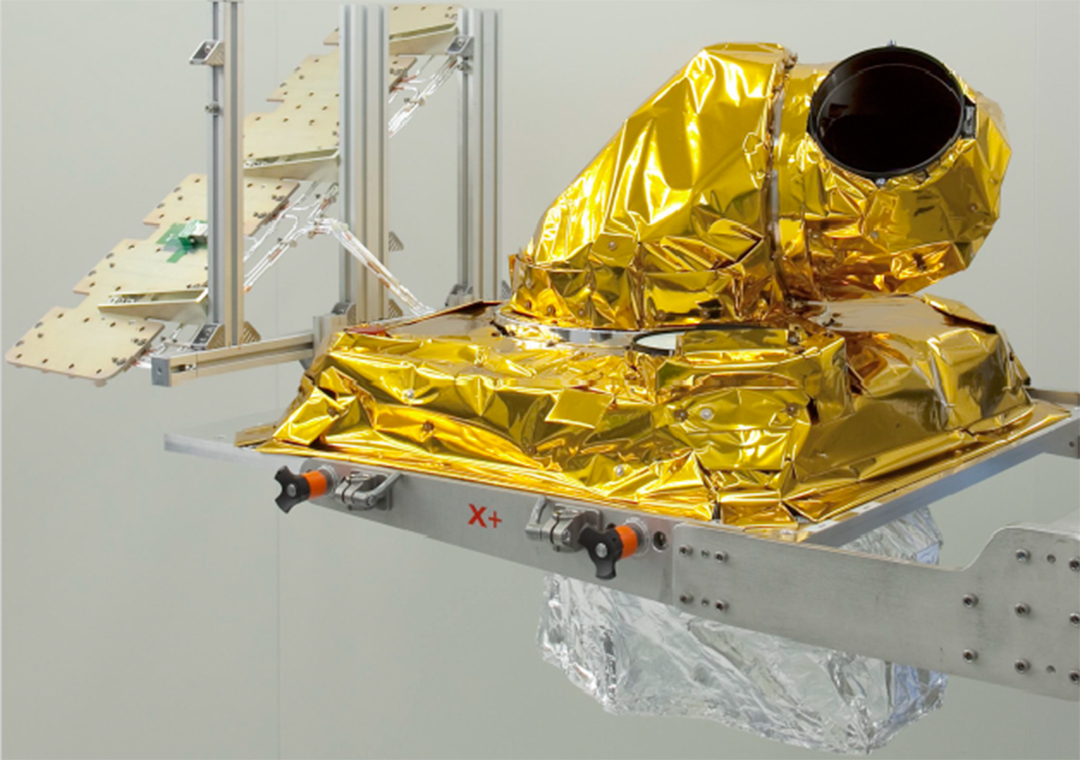
© ESA
To focus the laser beam, a telescope with a diameter of 13 centimetres only is
sufficient – across 42,000 kilometres, the light is so highly collimated that it can only be
received within a distance of a few hundred metres. The advantage is that even
a low transmission performance will do. Unauthorised “interception” is significantly
impeded. The reception of laser data transmissions on Earth is also possible: with the Transportable Optical Adaptive Optics Ground Station (TAOGS) from Tesat. This has been successfully tested with Alphasat. The advantage: a research institution on Earth – for example, a station in the Antarctic – can transmit data to a relay satellite via laser, from where the data are transmitted to a university to be evaluated.
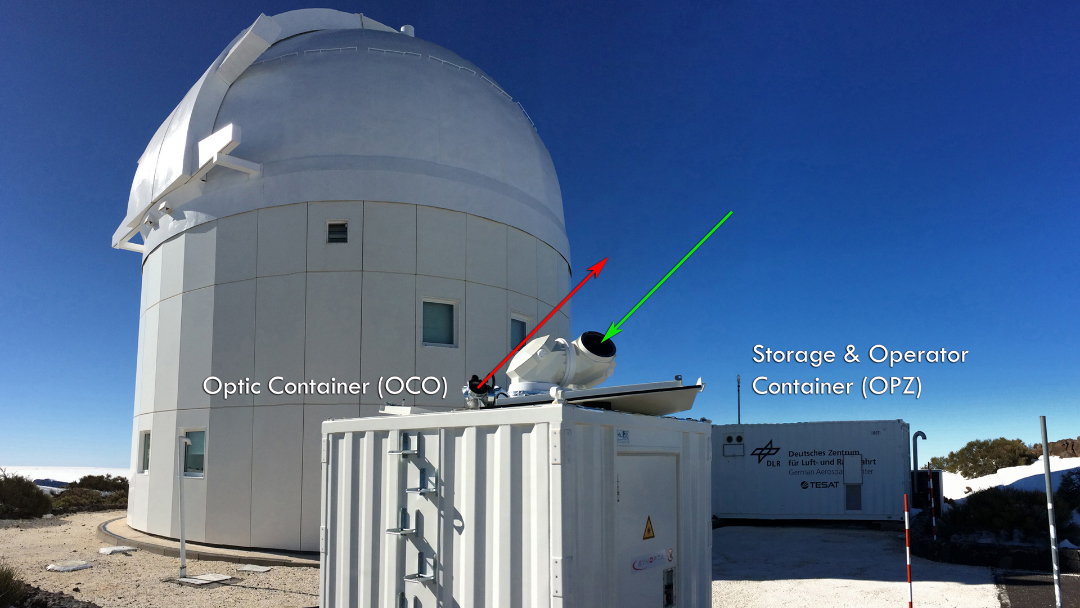
© Tesat-Spacecom GmbH & Co. KG - Transportable Adaptive Optical Ground Station at Observatorio del Teide (Tenerife, Spain)
And how fast is the internet
on the Moon?
Space probes produce enormous data volumes. Yet the communication with probes like, for example, Rosetta – launched in 2004 and arrived at the comet in 2014 – is based on radio communication. The transmission rate: 0.2 Mbit/s. This is very slow; however, it works over a distance of 455 million kilometres. Albeit it is inconceivable to think of video streaming.
© NASA
Laser actually works over really long distances. In any case, up to the Moon – that is almost 400,000 kilometres. In 2013, NASA succeeded in arriving at a transmission with 622 Mbit/s. This allows for the transmission of significantly better live video images than those of the first landing on the Moon. In the future, even laser connections to Mars are considered.
A short history on communication satellites
In 1858, a message from the United States needs ten days by ship to get to the United Kingdom. Telegraphy has already been invented, but it is no earlier than in this year that a transatlantic submarine cable can be laid. Now, a message can be transmitted within a few minutes. However, three weeks after, the cable is damaged and telegraphy becomes more reliable not until 1865.
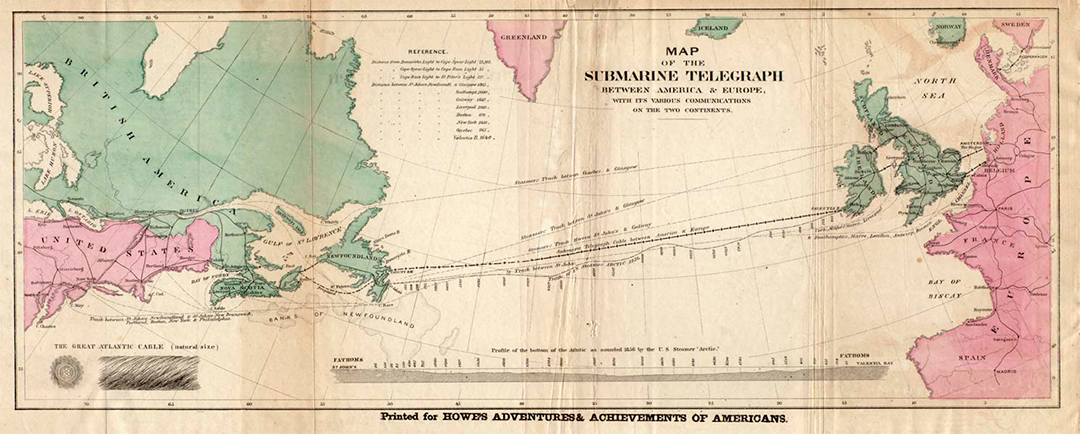
The route of the first transatlantic cables
© Wikipedia
In 1956, it gets easier to make phone calls between Europe and America. The submarine cable TAT-1 now enables 36 phone calls at the same time (among these also the direct connection by telex between the head of states in Washington and Moscow). For comparison: currently, the successor cables TAT-14 enable data rates of 3.2 Tbit/s, Emerald Express even 4 x 10 Tbit/s.
But how can animated pictures be transmitted from continent to continent – and that even live? Until 1962, the answer is: they can’t. The fastest way of “transmission” is to emplane a roll of film in an aircraft.
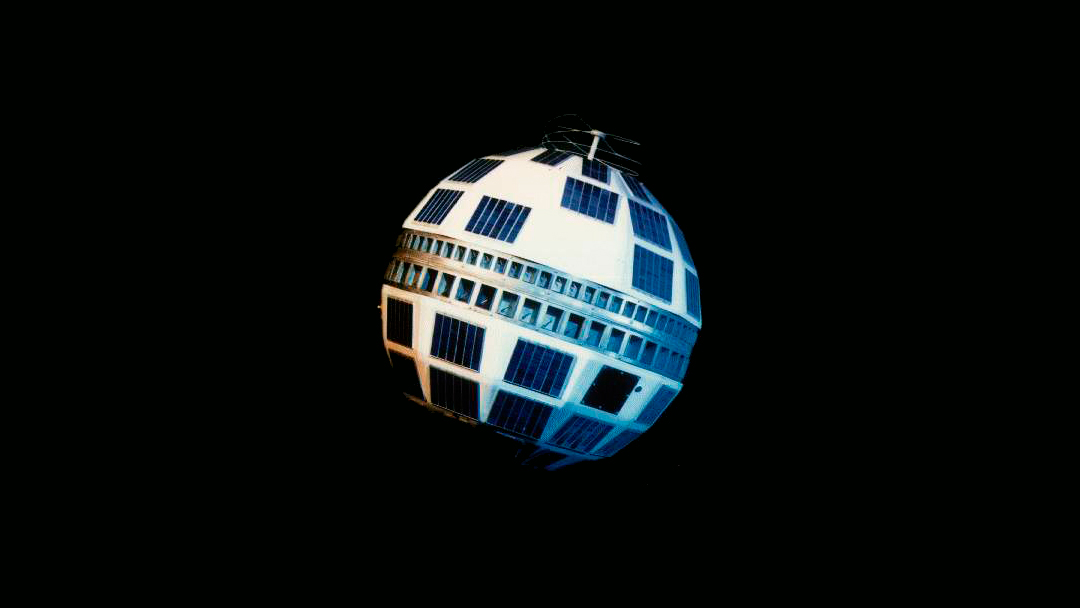
1962: Telstar, the first true communication satellite
© Wikipedia
This changes fundamentally with the 77-kilogramme light US TV and telephone satellite Telstar 1. For the first time, live images from the USA can be viewed in Europe and vice versa. They are received and transmitted from giant ground stations with antennas weighing 340 tons. The first TV programme via satellite reaches 200 million spectators in Europe.
© archive.org
However, since the satellite is not in a geostationary orbit, the connections last only
18 minutes. And the reliability leaves much to be desired as well. Half a year after the successful first transmission, the satellite fails because during launch, it gets into a particle cloud of a nuclear weapon test that has been ignited by the USA the day before.
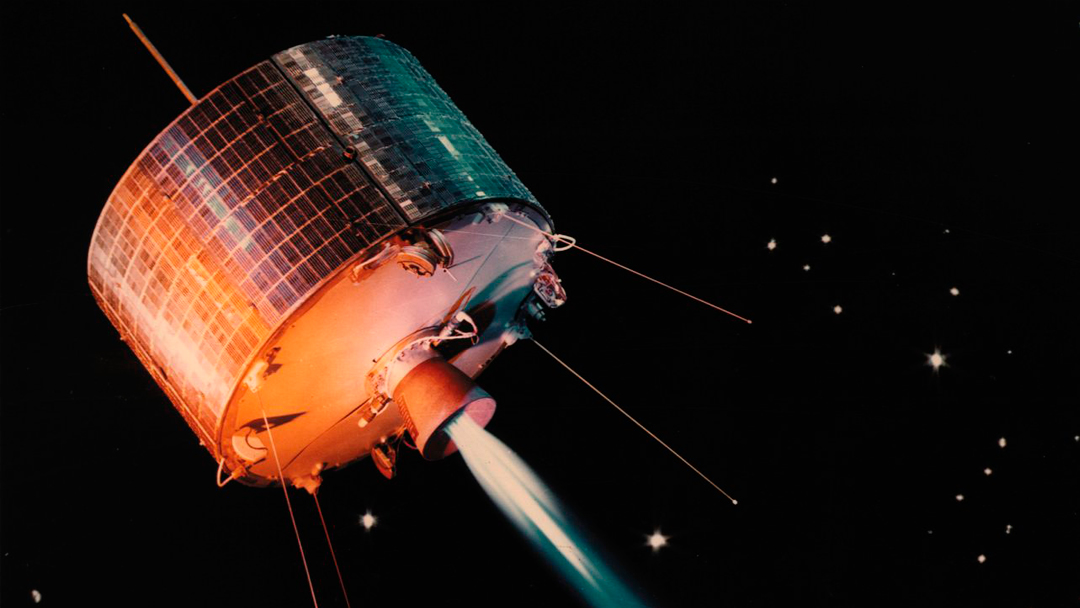
© NASA
A satellite that loses contact after a few minutes? Neither scientists nor TV makers are okay with that. Thanks to technical progress, in 1964 it is possible to broadcast the Olympic Games in Japan live to the US TV. In black and white and without sound (which came by phone) and in moderate quality, but anyhow: live. Now the transmission is done via the first geostationary communication satellite, Syncom 3. Since this satellite, viewed from Earth, is allegedly standing at one and the same point in space, connections without losing contact are now possible. Today, this is standard with communication satellites: with three geostationary satellites (plus the appertaining ground stations), a world-spanning communication network can be established.
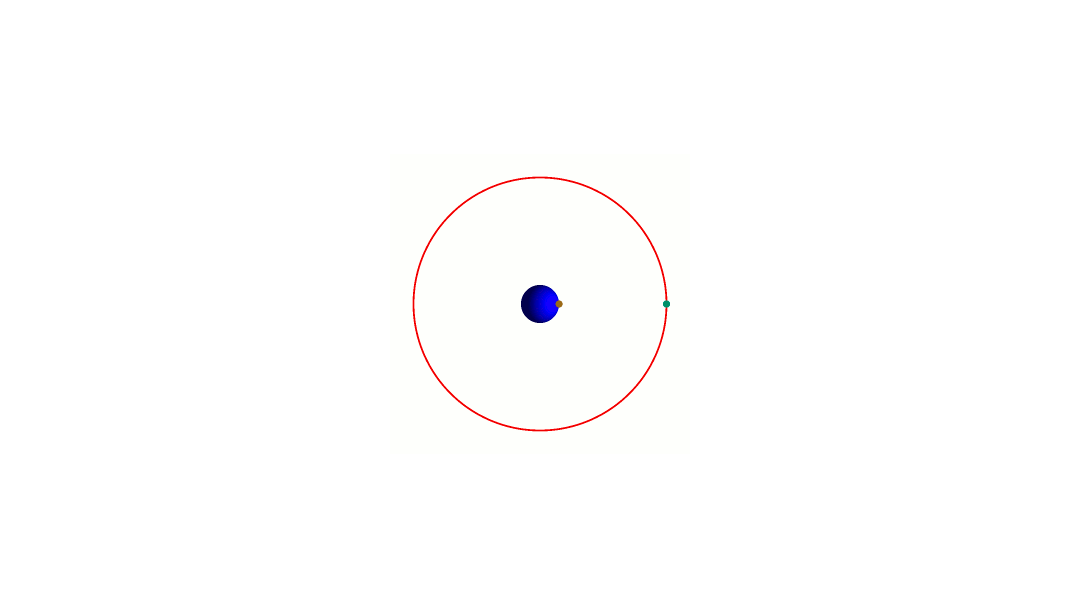
1969: Neil Armstrong’s historical steps on the Moon are broadcasted – live.
And all over the world. The broadcast that is viewed by 500 million spectators is successful, since less than three weeks before, with Intelsat III the first world-spanning network of geostationary communication satellites is put into operation.
© NASA
The astronauts broadcast from the Moon in black and white at ten images per second by means of a 66-cm sat dish via the S band (i.e. via frequencies that are used for satellite radio still today, even on board the ISS). The signals are received in the USA and Australia, transmitted to Houston via Intelsat where they are edited, and subsequently transmitted live to the whole world.
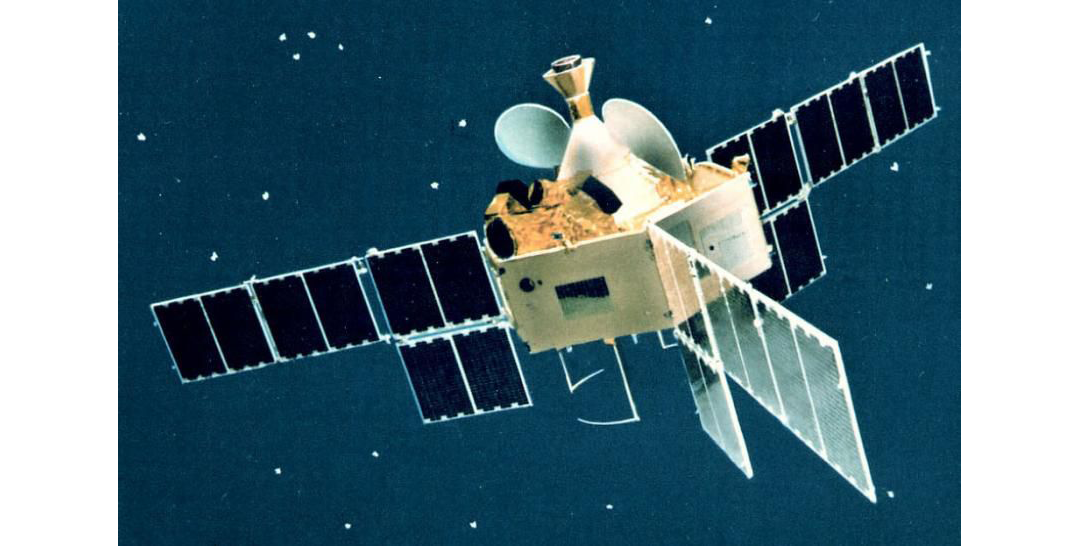
© Jean-Jacques Dechezelles, CC BY-SA 2.5
In 1974, the Franco-German satellite Symphonie is launched into space with two transponders on board.
In 1975, its identical sister module Symphony B is launched.
It is controlled by DLR Oberpfaffenhofen via their antennas in Raisting.
1975: While the Federal Republic of Germany with ARD (including its third programmes) and ZDF and the German Democratic Republic with DFF1 and DFF2
have only a few television stations, cable TV comes out on top in the USA. The
pay TV provider HBO manages to achieve the breakthrough, since thanks to
satellite technology (first on Weststar 1, thereafter on Satcom 1 with 24 transponders) now television can be broadcasted via several channels throughout the USA. As early as 1976, 120 transponders are existing on satellites for the USA – each transponder being capable of transmitting one television station. However, the customers of cable TV do not have a satellite dish on their roofs but receive the programmes via TV cable. The signals are transmitted to the local cable network from a ground station in the city (via satellite antennas).
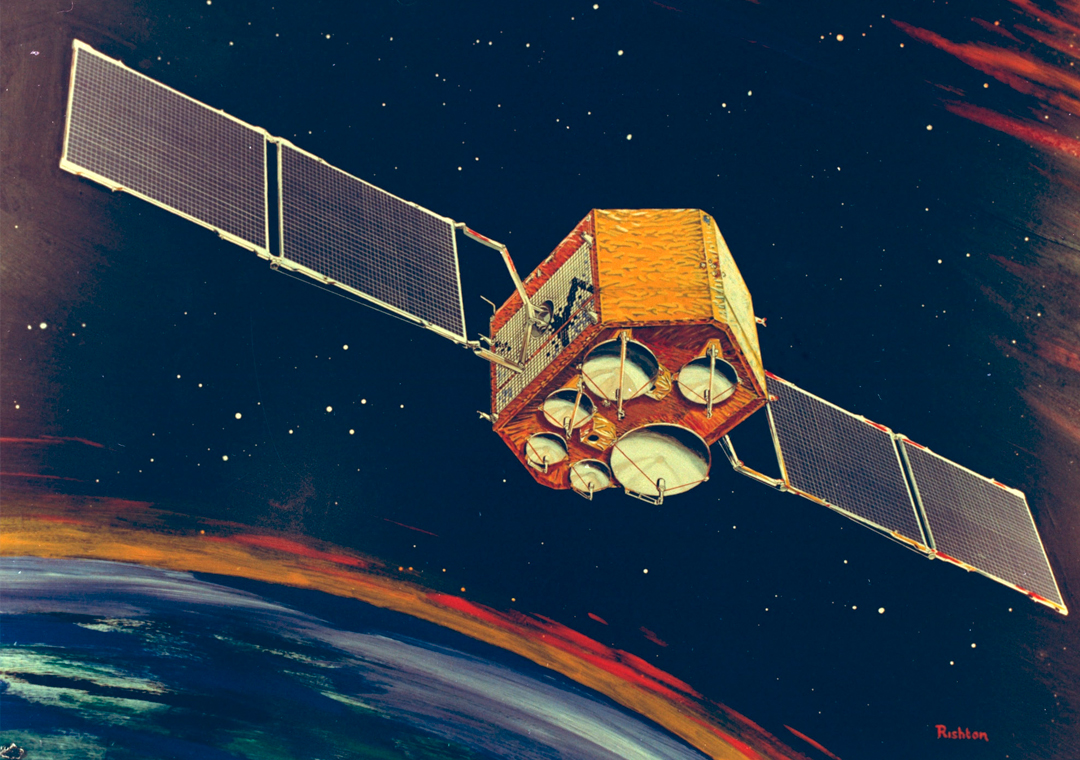
© ESA
In 1978, Europe follows: the European geostationary communication satellite OTS
with – after all – six transponders is launched. In 1982, the first sat TV television station becomes operational: Sky 1.
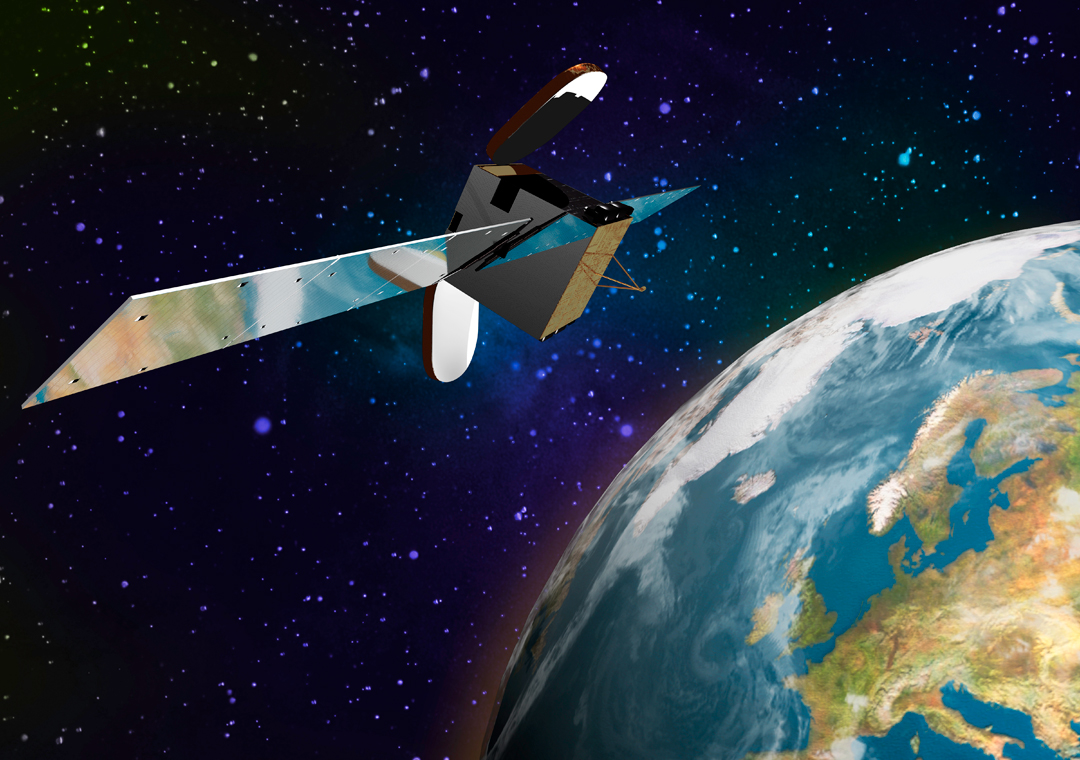
© SES
Starting 1988, Astra 1A is transmitting. With relatively small dishes (60–90 centimetres) and favourably priced receivers, 16 programmes can be received, among these, from 1989, the three most important private German television stations RTL, Sat.1, and Pro7. The Franco-German competition system TV-Sat is not able to come out on top although even dishes with a diameter of 45 centimetres are sufficient for reception. The reason: only four television stations can be transmitted, and even for these four special set-top boxes for the modern but exotic television standard D2-MAC are needed.
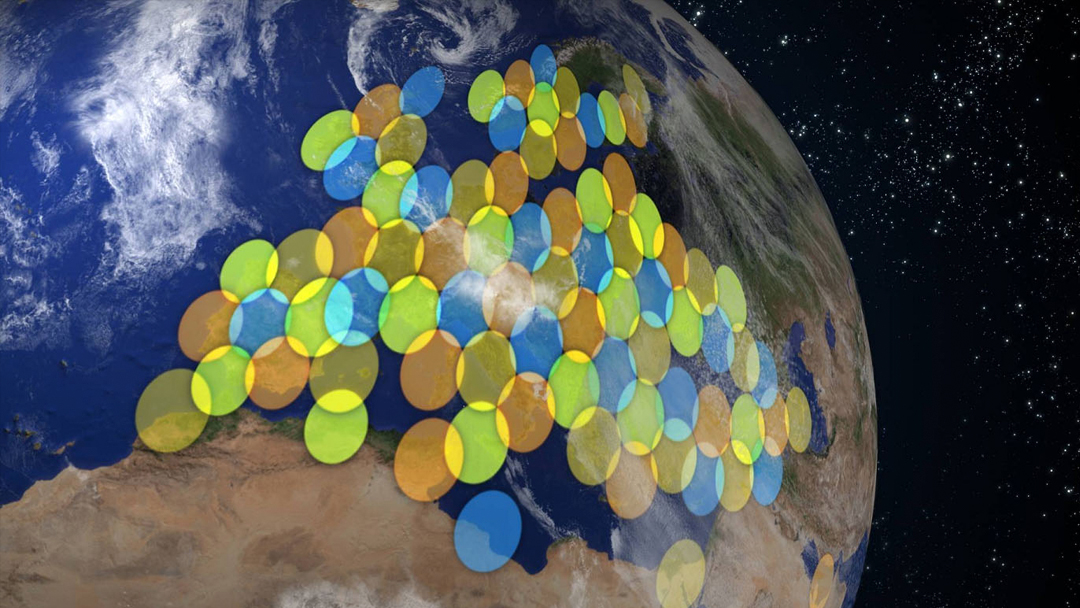
© Axlsite CC BY-SA 3.0
Television is still important today, however, not in its traditional shape: a firm and unalterable programme that all spectators are watching at the same time will soon be a thing of the past. Given the ever-increasing number of streaming offers and staggered viewing, fast internet connections get increasingly important from day to day – both for companies and private customers. Since 2010, Eutelsat KA-SAT has been providing Europe from 9° East with fast satellite internet. It has 82 transponders on board with a capacity of 475 Mbit/s each. Seven transponders are transmitting and receiving customer data from Germany; of course, the users have to share the maximum data rate of the transponder. This is why there are 22 Mbit/s available for each customer. Astra Connect with 20 Mbit/s provides an alternative.